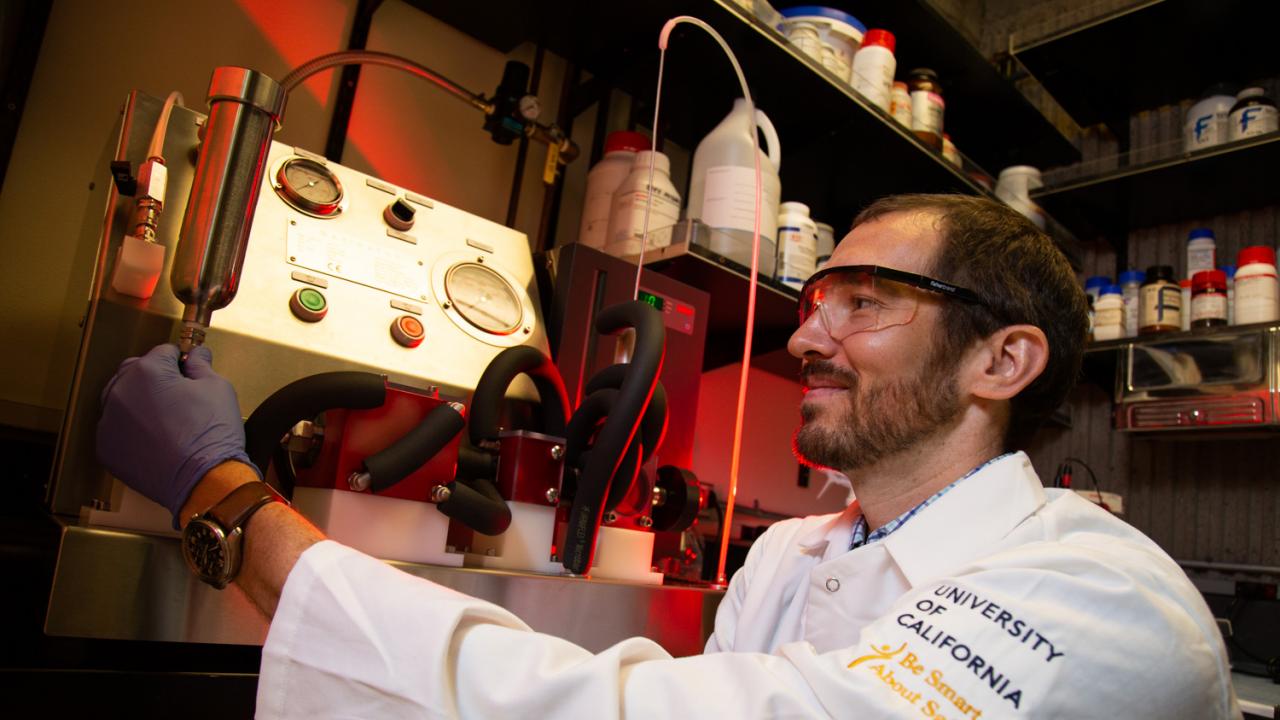
Mitochondrial Chitter-Chatter: Unveiling the Molecular Structures of Cellular Respiration
Quick Summary
- Dysfunction of the mitochondrial electron transport chain (ETC) is one of the leading causes of metabolic disease
- The ETC, which comprises four large membrane protein complexes, allows us to generate energy from the food we eat
- A new study published in Molecular Cell reveals the molecular structure of some of these complexes, uncovering further nuance in the relationship between them
In order to generate energy, our bodies transfer electrons from food—sugars, fats and proteins—to molecular oxygen, which allows our cells to respire and function. Performed by the mitochondrial electron transport chain (ETC), this process creates energy-storing and -transporting adenosine triphosphate (ATP), the “molecular currency” for energy in the cell.
“It’s a highly interconnected chain of events that basically provides you with this energy source in the end that can be used throughout the cell,” said Assistant Professor James Letts, Department of Molecular and Cellular Biology, of the ETC process leading to ATP. “That’s the main form of energy.”
The ETC comprises four large membrane protein complexes, which transfer electrons between one another via electron carrier molecules. In their natural membrane environment, these complexes come together in defined ratios to form what are called “supercomplexes.” Unstable outside of the cell, these supercomplexes and their molecular functionality have long been invisible, but new technologies are providing a glimpse of them.
In a Molecular Cell study, Letts and colleagues revealed that the function between the complexes in the respiratory supercomplex is more nuanced and variable than previously hypothesized.
“We are now for the first time able to purify a stable version of the supercomplex that’s functional,” said Letts. “We can now ask, “What is going on?” and ‘Why could it potentially be beneficial for bioenergetic metabolism?’”
Letts and colleagues found unexpectedly that two of the complexes in the respiratory supercomplex actively communicate, relaying information about their molecular structure to each other.
The research is unveiling interactions of the ETC at high resolutions, which could provide clues to how ETC dysfunction leads to disease.
“Dysfunction of the mitochondrial ETC is one of the leading causes of metabolic disease and largely the prognosis is poor,” said Letts. “In general, 50% of patients with ETC deficiencies die within the first two years of life and only 25% reach 10 years old. Despite this large medical need, currently there are no effective treatments.”
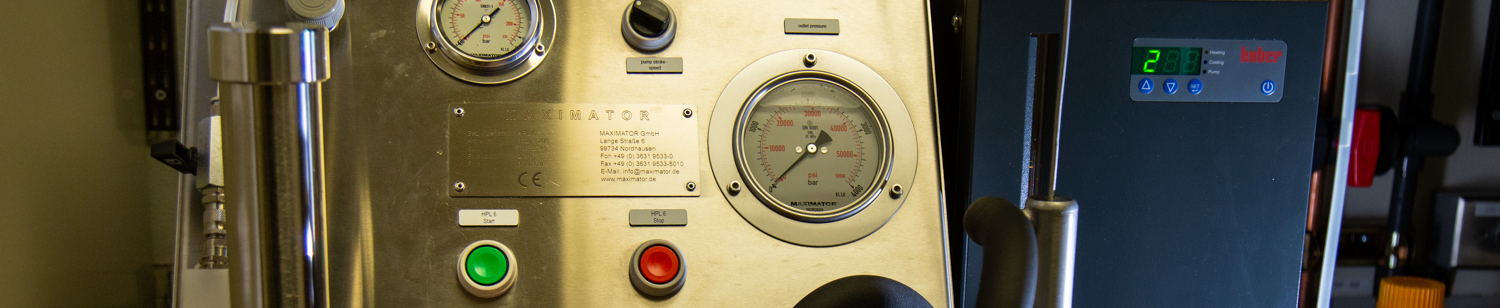
Protein complex, let my molecules go!
In the study, Letts and colleagues isolated the supercomplex made up of two of the four ETC complexes, known as complex I and complex III, from the cells of sheep hearts. The heart’s constant pumping requires a lot of energy, meaning its muscle tissue is brimming with mitochondria. The red color of the heart is actually due to the high amount of mitochondria.
A previous hypothesis suggested that, when bound together in the supercomplex, complex I and complex III shuttle ubiquinone molecules—essential to energy transfer—back and forth in a trapped manner.
“If they trap ubiquinone between complex I and complex III and prevent it from exchanging with the broader ubiquinone pool in the membrane it might allow for quicker electron transfer overall,” said Letts, explaining the previous hypothesis. “There are other enzyme systems where this is the case.”
When the team trapped ubiquinone between the complexes in their assays, however, they found the overall rate of the system declined. “Complex III becomes rate limiting and therefore complex I can’t turn over as fast as it could if ubiquinone was free to exchange with the broader pool,” said Letts.
When the team freed the ubiquinone, the supercomplex performed much faster, showing that trapping ubiquinone decreases the rate of electron transfer and disproving the previous hypothesis.
A technique called single-particle cryo-electron microscopy gave the team an unprecedented look at the structure of complex I and complex III together. This allowed them to create 3-D reconstructions of the molecular structures.
“One thing we’re able to do with electron microscopy maps is we’re able to look at what’s called local resolution and that’s a measure of the flexibility of different regions of the complexes,” said Letts.
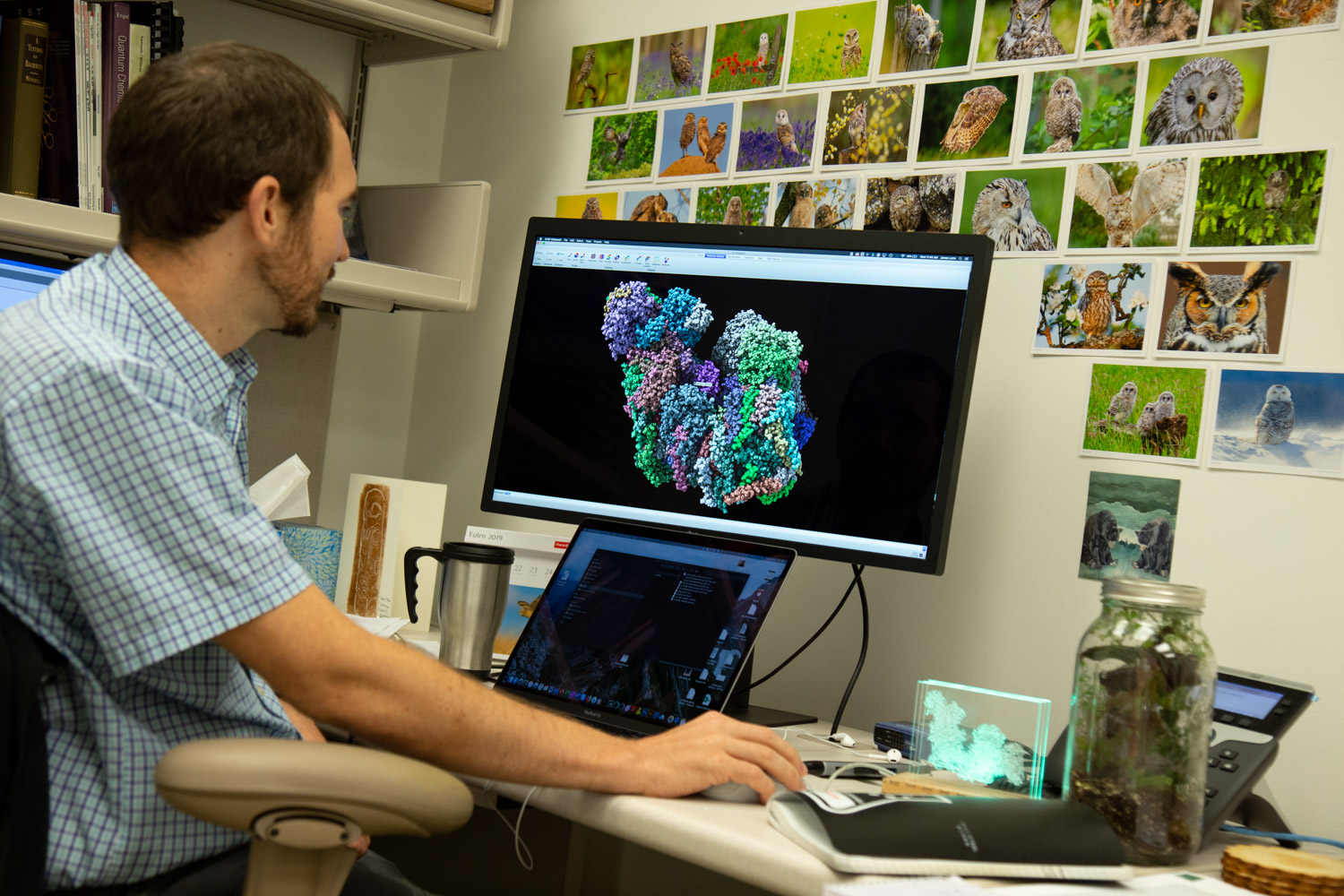
Unexpected crosstalk between regions
The team also found that complex I exhibited “open” and “closed” conformations and that these conformations affected the functionality and conformation of complex III. Based on the experiments, the team solved four different structures of the respiratory supercomplex.
One of the standout findings was that complex I can affect the symmetry of complex III, which is a dimer, meaning it’s made from two identical proteins. Complex III has four ubiquinone binding sites. The researchers found that when complex III was attached to complex I, the ubiquinone binding site on complex III closest to complex I, unlike the other three sites, did not have ubiquinone bound.
“There seems to be functionally relevant symmetry breaking of complex III,” said Letts.
The findings indicate that there’s crosstalk between complex I and complex III. Letts said the asymmetric ubiquinone binding site could be interacting with complex I somehow.
“Complex III is talking to complex I essentially and complex I is talking to complex III and they’re telling each other about what catalytic states they’re in,” he said. “We don’t exactly understand how that communication is going on but complex III clearly can sense the state of complex I based on this data.”
“It’s really remarkable,” he added. “Nobody had predicted such a nuanced role for these supercomplexes. It makes us have to rethink everything we thought we knew about how they work and why they evolved.”
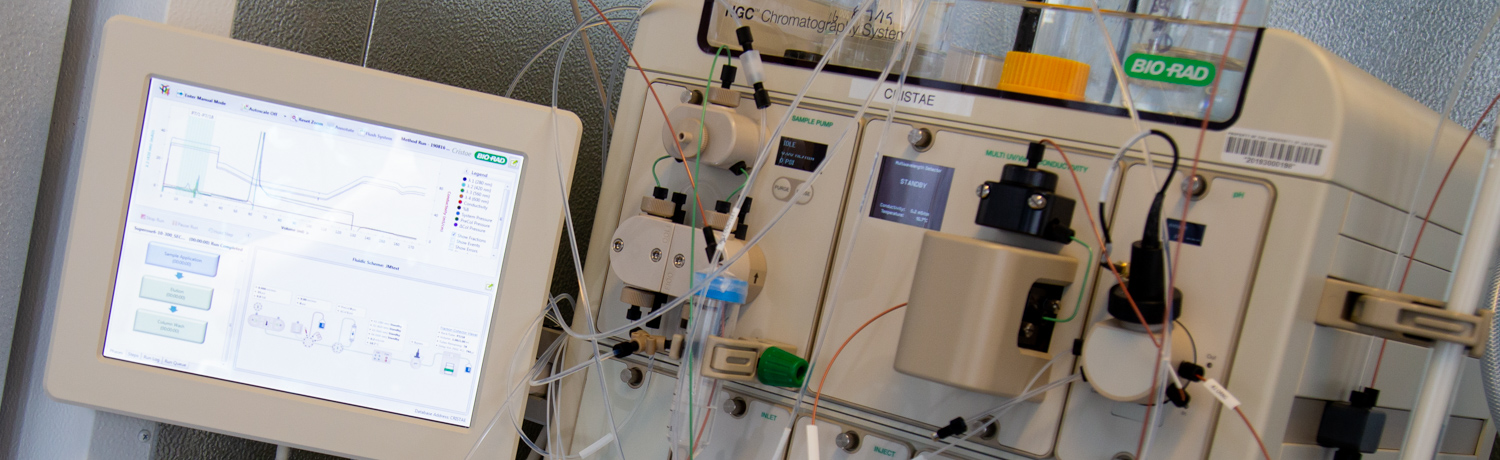
Detergents? Who needs ‘em?
One of the difficult things about working with membrane proteins, like complex I and complex III, is they’re hydrophobic, or repelled by water. They prefer oily membrane-like environments, like their home inside the cell. Outside of the cell membrane, they clump together and are useless. To work with membrane proteins in the lab, molecular biologists usually use detergents, which work similarly to laundry detergents.
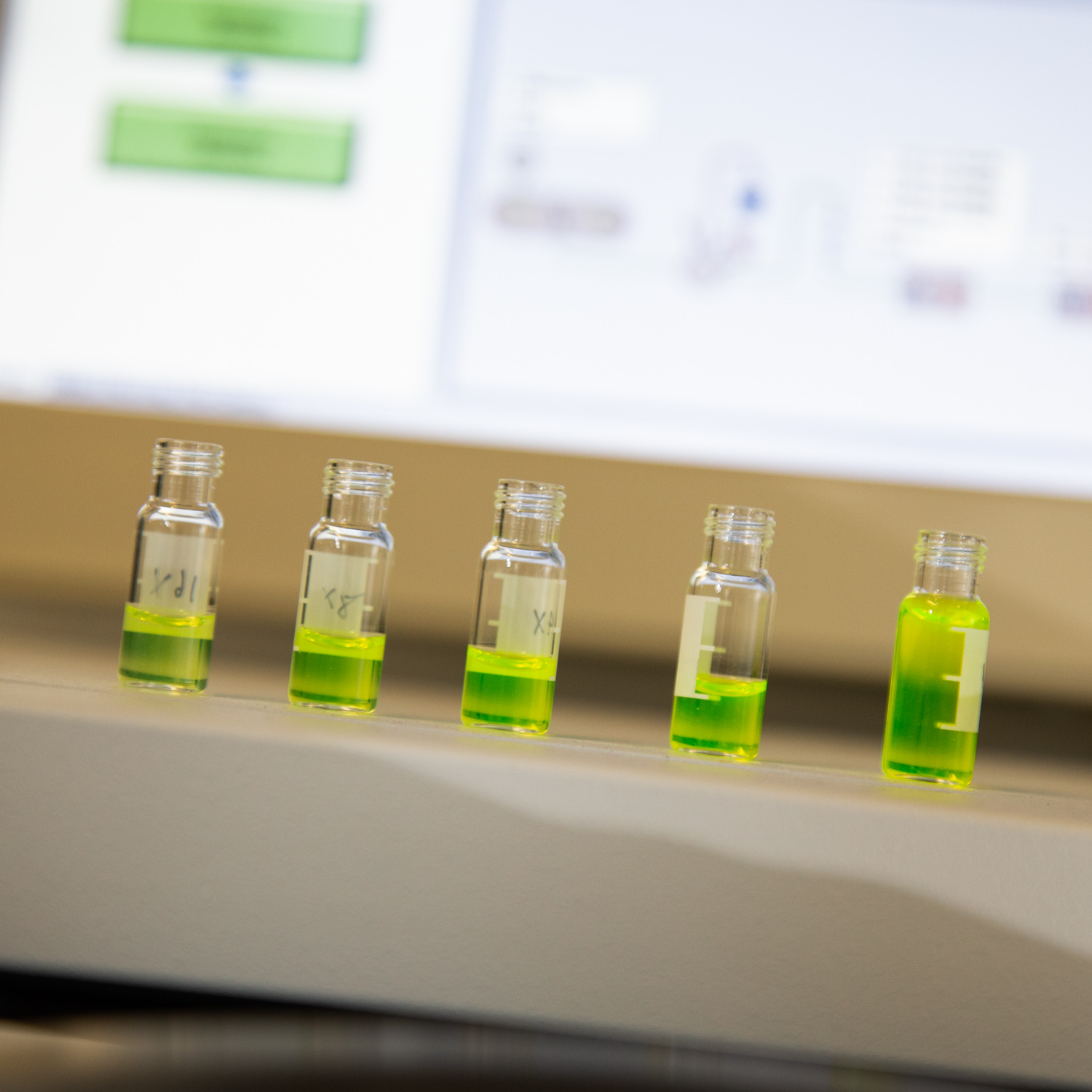
“They’re obviously a little more sophisticated, but it’s basically the same thing,” said Letts. “They go into the membrane and they stabilize the hydrophobic parts of the membrane protein and allow it to come into solution so that you can work with it in solution.”
While advantageous, these detergents can also get in the way of science, sometimes making it difficult to perform activity assays r glean molecular structures.
Letts bypassed these molecular hiccups in the Molecular Cell study by using amphipols, a polymer that can wrap around membrane proteins and stabilize them in a solution.
“You can remove all the detergent that normally you need around to keep it soluble,” said Letts. “That’s what we were able to do with this supercomplex.”
“This is the first time anyone has ever been able to purify it,” he added.
The research was supported by the European Research Council through the Marie Skłodowska Curie Individual Fellowship.
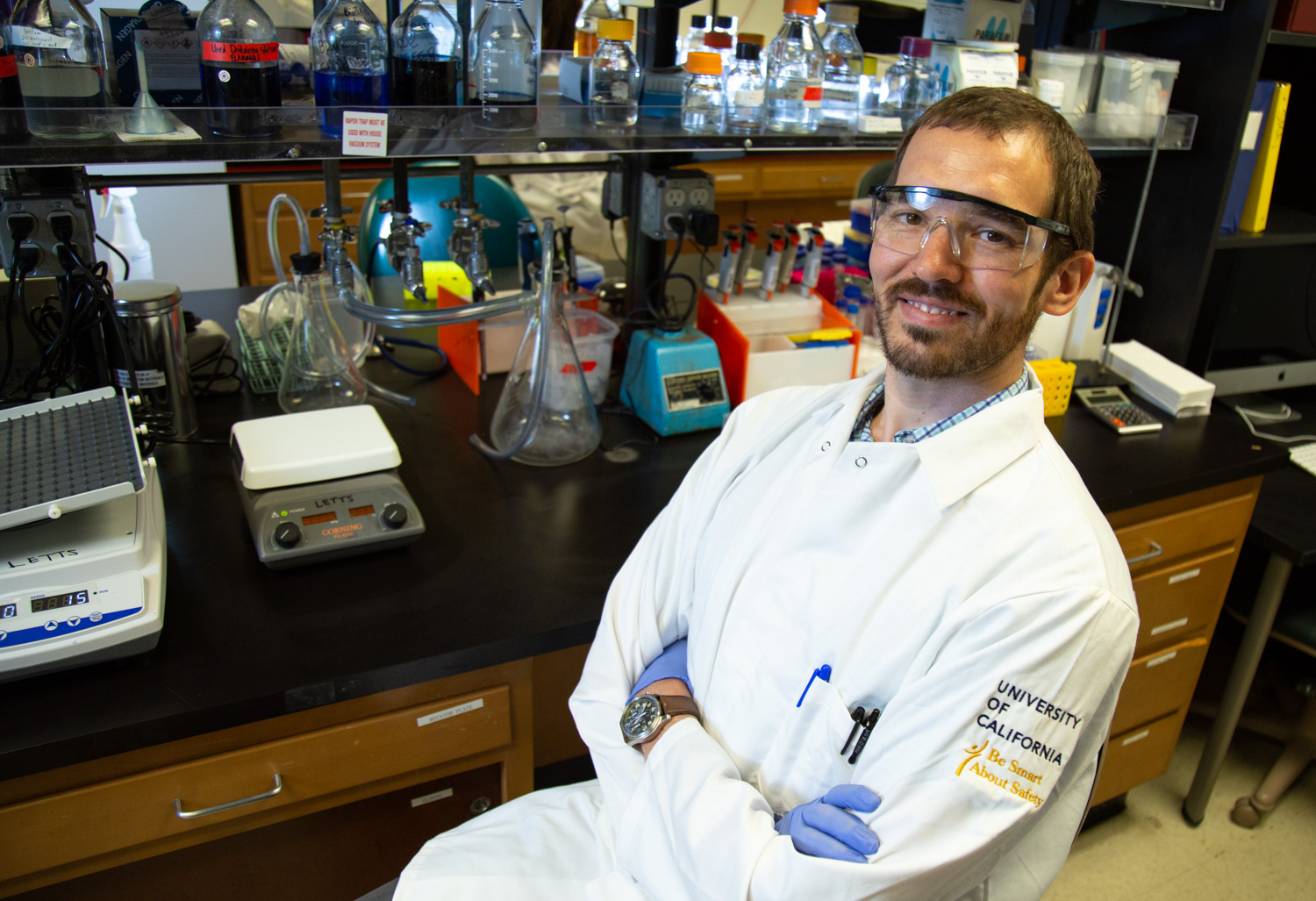