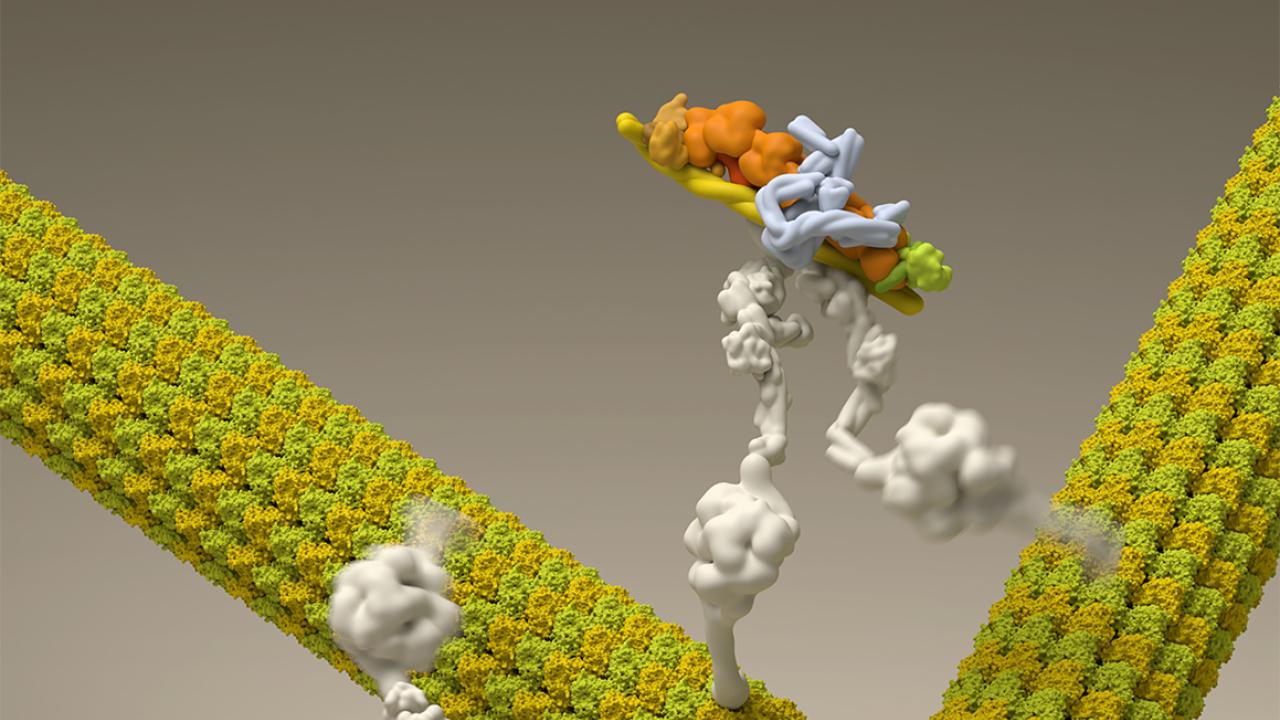
Understanding Traffic Congestion on the Shifting Roadways of Cell Division
Within every cell is a transportation system that rivals our most complex roadways and interchanges. Known collectively as the cytoskeleton, this system is used by molecular machines known as motor proteins to transport numerous types of cellular cargoes throughout the cell.
In a new study appearing in Developmental Cell, Ruensern Tan, a biochemistry, molecular, cellular and developmental biology graduate student, and Assistant Professor Richard McKenney, Department of Molecular and Cellular Biology, provide a new molecular model to describe the role of a motor protein called cytoplasmic dynein in restructuring the cytoskeleton for cell division. Identifying problems in this process is vital to understanding the origin of many diseases.
“This is a very fundamental process that occurs in all the cells in your body,” said McKenney. “When defects occur in this process, you get defects in chromosome segregation, which is one of the triggers for cancer development.”
A clustering of proteins
Of all the motor proteins used for intracellular transport, dynein is the most versatile in its functionality. Like a cargo truck, it transports materials throughout the cell, but it also plays a role in reshaping the cell’s cytoskeletal structures. One major restructuring occurs during cell division.
Within the cytoskeleton, dynein travels on hollow cylindrical roads called microtubules. Dynein moves in one direction towards what is known as the microtubule’s minus end.
During cell division, microtubules dramatically rearrange to form the mitotic spindle, a football-shaped molecular machine that moves chromosomes to both of its ends in preparation for the division of the cell, known as mitosis.
Using a technique called single-molecule total-internal reflection fluorescence microscopy, Tan and McKenney were able to directly observe purified microtubules and motor proteins engaging in this process outside of living cells.
“When dyneins reach the end of the microtubule track, they actually tend to accumulate there,” said McKenney. “We thought this was a curious behavior because we naively guessed they would fall off upon reaching the end of the microtubule.”
When microtubules with an accumulation of dyneins congregate, the dyneins hop onto an adjacent microtubule while maintaining a grip on their original microtubule. It’s as if they’re driving on two roads at the same time. The force resulting from this action morphs the various microtubules into a single structure, which Tan and McKenney termed a “microtubule aster.”
According to McKenney, this process is reminiscent of what occurs in living cells during mitosis, where the mitotic spindle is built via the assembly of such microtubule asters. This critical process is necessary to divide the cell’s genetic information and ensure this information is equally transferred to both new cells produced from mitosis.
“We can actually see this happening with microtubules and dynein in a purified system, and we feel very confident that these observations provide a likely model for what is happening in cells,” said McKenney. “In cells, it’s fairly clear that there are other molecules involved and we think those molecules might play regulatory roles, but our study suggests when it comes to the mechanical work, dynein can accomplish the task alone.”
Implications for diseases
Complications in the formation of the mitotic spindle are of great interest to cancer researchers since cancer is a disease of cell division. But Tan and McKenney’s molecular model could also be applied to other diseases.
“It is clear that impairment of intracellular transport leads to developmental and neurological disorders,” said Tan. “As we move forward with our understanding of intracellular transport, we will be able to harness this power to provide a healthier tomorrow.”
The study was performed in collaboration with researchers from Harvard University’s Department of Molecular and Cellular Biology and the FAS Center for Systems Biology. Funding was provided by the National Institutes of General Medical Sciences.